Sludge and circularity − a report on the 8th Conference of the UK Wastewater Network
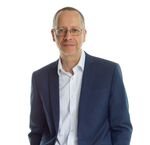
The Conference of the UK Wastewater Network is an annual one-day conference hosted by Cranfield Water Science Institute of Cranfield University in the UK.
This year, due to coronavirus, the 8th Conference of the UK Wastewater Network was held online for the first time, on 26 November 2020. Simon Judd reports on the conference's succinct but eclectic programme which featured presentations from the UK, Belgium and Australia with a circular economy focus
The programme of the 8th Conference of the UK Wastewater Network focused on the circular economy, with talks on resource recovery (microbial protein and coagulant), ammonia to energy conversion, biosolids to land carbon accounting, as well as an examination of microplastics impacts.
1. Iron coagulant reuse and recovery
Kicking off with iron coagulant reuse and recovery, Dr Ilje Pikaar from the University of Queensland (presenting his work at 8:00 PM local time) made a strong case for substituting alum coagulant with iron to allow its reuse and possibly reclamation. The idea is to use ferric coagulant for drinking water treatment, and then use the ferric-rich sludge generated in place of the virgin coagulant reagent for further duties.

Pikaar demonstrated surprising efficacy from dosing of the drinking water sludge (DWS) directly into the sewer network. This has two beneficial impacts:
- suppression of hydrogen sulphide (H2S) generated in the network, and
- the subsequent precipitation of iron phosphate as vivianite.
The use of ferric for H2S mitigation is well-established: it’s fairly widely used in regions such as Australia where the warm wastewater temperatures combine with septicity to generate H2S in the sewer network. However, substituting ferric chloride with potable sludge is quite a leap.
A further step was then made in closing the loop: recovering the iron from the wastewater sludge as iron phosphate in the form of vivianite. Crystalline vivianite is a naturally-occurring gemstone, with a lustrous green colour. Hard to imagine it being recovered from sewage sludge.
The group has successfully demonstrated this concept during long-term laboratory scale testing – an impressive feat, given that iron chemistry is something of a black art. There are more aqueous iron species than you can shake a stick at.
The trials were conducted based on continuous flow systems which simulated an urban wastewater scheme comprising sewers, wastewater treatment reactors, sludge thickeners, and anaerobic sludge digesters. The results indicate that the sludge performs almost as well as the virgin ferric chloride, and that most of the iron ends up as vivianite (Fig. 2). The vivianite could then be recovered using magnetic separation, as used in mining industry.

Two further presentations provided a perspective on the impact of biosolids application to land. The first of these, from Dave Spurgeon of the UK Centre for Ecology and Hydrology, focused on the dangers of microplastics in soil systems through a consideration of exposure, risks and impacts.
2. Biosolids to land
There are, as pointed out by the presenter Dave Spurgeon, a plethora of papers on the measurement of microplastics (MPs) in water – in particular the marine environment. The water can be filtered and the plastic material sieved out for further analysis. It’s a tad more challenging to extract plastic from soil and sludge. Methods used comprise advanced oxidation followed by flotation, then enzyme digestion, and then dispersion in ethanol for analysis by Fourier transform infrared (FTIR) and/or pyrolysis GC-MS.
Using such analyses, the MP content of soil receiving biosolids has been shown to be at least measurable. One study has indicated that for biosolids application at a rate of 250 kg of N per hectare per yr, equating to 3 g sludge/kg soil, around 30,000 microplastics particles per kg of soil can be detected. Across different countries, reported mean concentrations of MPs in sewage sludge have ranged from below one to over a hundred particles per g dry weight (Fig. 3).
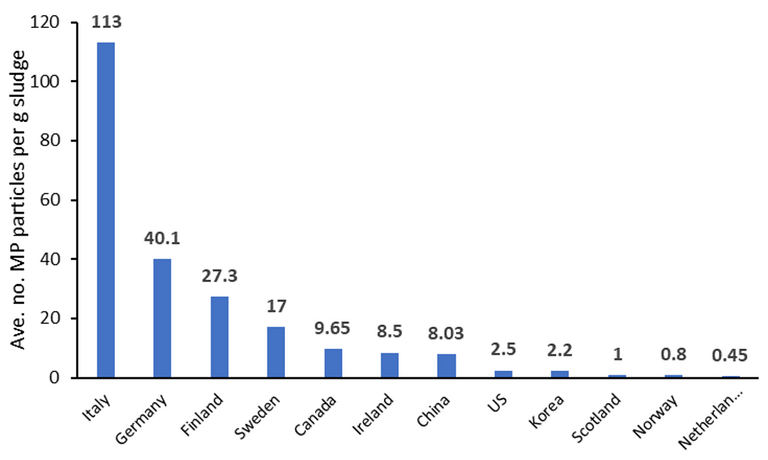
As Spurgeon acknowledges, it’s difficult to know how significant these concentrations are long-term, or even whether the detected microplastics derive from the biosolids rather than from litter. From a water company and regulator perspective, any detected microplastic is clearly undesirable. The group at CEH have established that, with respect to impact on worms specifically, reproduction is significantly affected at higher concentrations (> 90 g/kg soil in the case of nylon).
In the case of nanoplastics (100 nm diameter, as opposed to microplastics of around 0.1 mm), detection is solid and sludge becomes even more challenging. In terms of impacts, outcomes suggest that positively charged nanoplastic particles negatively impact on worm mortality.
The second presentation on biosolids to land was from Andy McLeod of Jacobs Engineering. This work, in which the positive benefits of employing biosolids for soil amendment were demonstrated through carbon accounting (Fig. 4), has already been outlined elsewhere (see our feature UK water net zero carbon: the true benefit of biosolids to land). This work addresses the mostly overlooked component of carbon sequestration by the soil, rather than the more widely reported release of carbon and greenhouse gases to the atmosphere.

Several interesting outcomes were presented, including the impact of sludge processing on the extent to which the organic carbon is retained in the soil. It appears that sludge drying may promote the loss of organic carbon more than dewatering or composting.
Next, two intriguing presentations were presented concerning the fate of ammonia, and its significance as a resource. Two quite different reuse opportunities were considered.
3. Ammonia recovery for microbial protein
Korneel Rabaey of Ghent University introduced the audience to the exotic world of microbial protein (MP). It is very widely acknowledged that the classical method for producing protein (i.e. meat) is a highly inefficient way of converting nitrogen to protein (or, indeed, for using water). Only about 4% of the nitrogen applied to the process ends up as protein in the product. For plant-based protein products it’s substantially better, but still only 15%. But if we were all to start eating microbial proteins, the figure would jump up to 46%.
MPs are rich in essential nutrients – more so than plant-based proteins, such as soya. They can be adapted to the olfactory systems of the target species (humans, for example, discern odours somewhat differently to, say, pigs). They require a lot less land and a lot less water than other protein sources.
So, what’s all this got to do with wastewater? The answer is: nitrogen.
Sewage is a pretty decent source of ammonia – and some manure sources (pigs, again) especially so. All microbes require both carbon and nitrogen. The carbon can be harnessed from the anaerobic digestion biogas, either directly from the methane (Fig. 5, Case 1) or from the CO2
downstream of the CHP (Fig. 5, Case 2). If the ammonia can also be extracted from the AD digestate supernatant, then the protein can be generated from a sustainable source of both C and N.
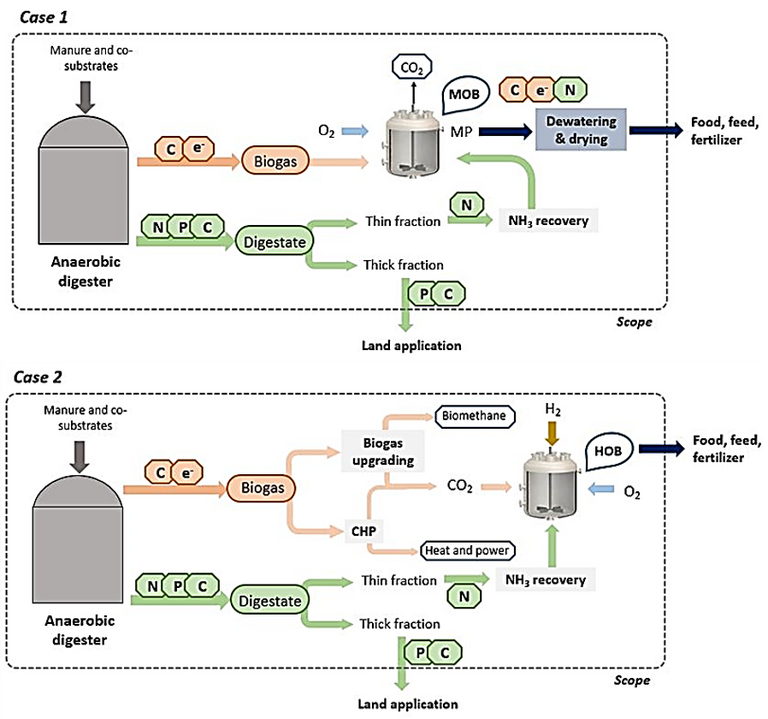
There is a certain elegance in this. If the microbial protein produced from a pig farm effluent ammonia source were to be used as animal feed then, apparently, about one third of the protein needed to sustain the pig can be provided by its own effluent. Probably best they don’t know.
All this points to the importance of uber-efficient solid-liquid separation combined with appropriate treatment of the supernatant from the anaerobic digester (Fig. 5). The conventional approach is to return this ammonia-laden stream to the head of works. It seems that this is criminal waste of both ammonia and energy.
4. Ammonia recovery for energy
The final presentation, from Ewan McAdam at Cranfield University, also addressed the value of ammonia, in this instance for generating energy.
Clearly, the ammonia economy is somewhat lower in profile than the hydrogen economy. Hydrogen appears attractive on the basis of its calorific value per unit mass (120 MJ/kg – considerably higher than the corresponding figure of 22.5 MJ/kg for ammonia). However, crucially, ammonia can be readily liquified. This means its CV per unit volume is around 17 MJ/m3 – actually more than the 11 MJ/m3 for H2 at STP. Ammonia is also less hazardous to transport.
The current practice of using microbiology to convert ammonia to nitrate accounts for more than a quarter of the 0.63 kWh per m3 of municipal wastewater treated by the conventional activated sludge process. If instead this ammonia is captured directly it can potentially generate 0.19 kWh/m3.
Indeed, it is already the case that combined heat and power (CHP) engines can be adapted to run on ammonia, or a combined biogas/ammonia feed. Ammonia can also be electrolysed to generate hydrogen.
As with Rabaey’s presentation, the key is to capture the ammonia at the point at which it’s concentration is the highest in the wastewater treatment process. In the scheme presented by McAdam (Fig. 6), an anaerobic stage is applied to both the wastewater treatment and sludge streams, with ammonia captured from the anaerobic MBR offgas and from the centrate of the dewatering centrifuge treating the AD sludge.

The technology for ammonia capture is not without challenges. Ammonium has to be converted to ammonia by raising the pH. Ammonia stripping is more effective at elevated temperatures. There are surface fouling issues in the stripper. Finally, the gases have to be concentrated and purified for end use. However, there is potential energy to spare thanks to obviating the biochemical nitrification step, and an associated environmental impact benefit through avoiding the emission of N2O – a powerful greenhouse gas.
5. Summary
It seems there’s a lot of good that can come from sludge, or the processes associated with it.
Direct application of the sludge to land offers benefits not only from the nutrients delivered but also in terms of holistic carbon accounting. This relies on retention of the organic carbon in the soil, rather than its release into the atmosphere.
It appears possible to use potable water sludge, generated from dosing with ferric coagulant, to use as an alternative to the coagulant itself. Application directly to the sewer network both suppresses hydrogen sulphide and encourages precipitation of ferric phosphate (as vivianite) in the sludge generated from the downstream wastewater treatment processes.
Finally, there is the intriguing issue of ammonia. The classical approach to ammoniacal species is to biologically convert them to nitrate and then nitrogen to render it harmless. However, ammonia itself is a useful resource for energy and protein production. Ironically, perhaps the greatest impediment to implementing both of these options is critical mass: there needs to be enough ammonia generated in a single location to offset the associated capital expenditure for its reuse. However, ammonia is at least reasonably transportable as a pressurised liquid.
True, there is justifiable concern over the levels of substantially non-biodegradable and potentially harmful contaminants, specifically microplastics and the derived products such as polyfluoroalkyl substances (PFAS). The presence of these substances in biosolids applied to soil presents a massive challenge which, it seems, can only realistically be met by source control.
However, the remorseless and essential drive towards the circular economy makes it inevitable that some or all of these ideas will ultimately be implemented at a substantial scale. Not everyone is going to rejoice in the idea of tucking into a slab of pork or veggie meat which has used a waste stream from a sewage treatment works as a food source, and reusing, repurposing and/or reprocessing potable water sludge presents significant logistical challenges. Ultimately, though, the only limitation is commitment.