Protect and survive: the fate of pathogens in stored sludge
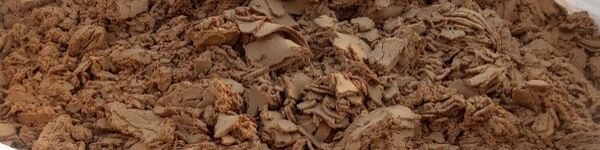
S. Fane 1, P. Vale2, Y. Bajon-Fernandez1, E. Cartmell3, A. Nocker4, J. Harris1 and S. Tyrrel1
1 Cranfield Water Science Institute, Cranfield University, Cranfield, MK43 0AL
2 Severn Trent Plc., UK
3 Scottish Water, Dunfermline, Scotland
4 IWW Rheinisch-Westfälisches Institut für Wasserforschung gemeinnützige GmbH, Germany
Corresponding Author: s.tyrrel@cranfield.ac.uk
1. Introduction
Increasing emphasis on the circular economy and extracting value from waste has focused increased attention on the pathogen content of biosolids (i.e. treated wastewater sludge) intended for reuse in agriculture, based primarily on E. coli as an indicator micro-organism. International guidelines (Table 1) in this regard are based either on bacterial concentration or minimum log removal from treatment.
Table 1. International microbial compliance levels required/proposed for treated biosolids for agricultural use
Table 1. International microbial compliance levels required/proposed for treated biosolids for agricultural use
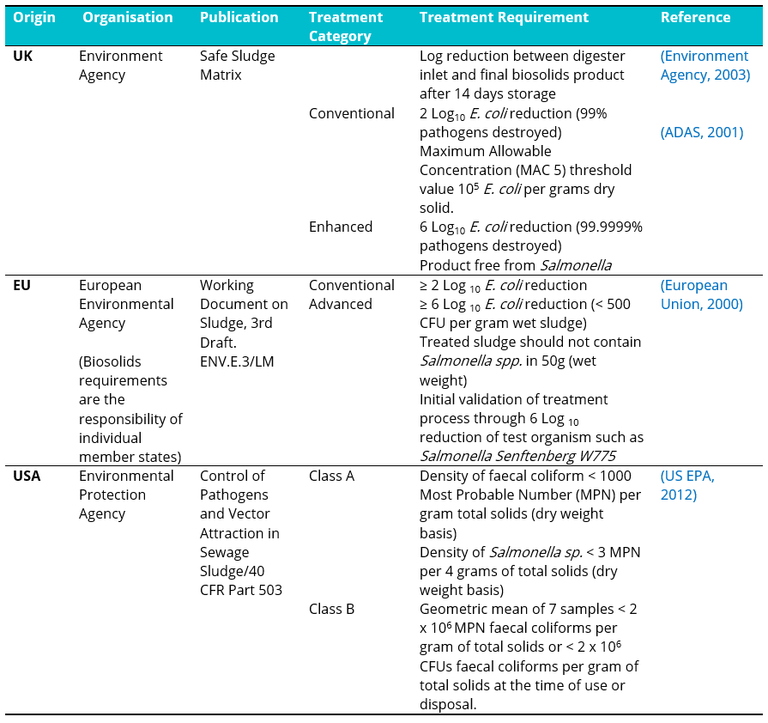
Whilst dewatering operations tend to increase pathogen levels in the cake product, by up to six orders of magnitude in the case of centrifugal dewatering when compared to the feed (Qi et al., 2007), this is countered by anaerobic digestion (AD). However, levels in the dewatered cake appear to be highly sensitive to storage conditions and time, both for a raw and digested sludge feed (Higgins et al., 2007(a)). Clearly, it is worthwhile considering these conditions further to try and understand how the growth of pathogens can be mitigated.
2. Dewatering shear effects and polyelectrolyte dosing
It has been suggested that polyelectrolyte dosing upstream of mechanical dewatering of AD sludge may promote bacterial growth by trapping bioavailable protein and polysaccharide within the flocs (Monteleone et al., 2004). Floc formation may also protect bacterial cells from environmental stressors, prolonging cell survival (Lin et al., 2014; Mahendran et al., 2012).
High shears created by centrifugation can cause floc breakage and subsequent dispersal of bacteria and bioavailable organic matter (BOM). It has been suggested that it is this BOM substrate which promotes pathogen regrowth (Chen et al., 2011; Monteleone et al., 2004; Qi et al., 2008, 2007), since regrowth has been shown to relate to the applied shear (Chen et al., 2011). It is also possible for shear to lyse some of the cells themselves, providing additional bioavailable protein and nutrients for growth of the remaining bacterial population (Sun et al., 2015).
However, the substantial increases in indicator pathogen levels reported following sludge dewatering cannot be attributed to growth alone, since the E. coli doubling time is 20 minutes even under optimal conditions (Higgins et al., 2007(a)) − about the same as centrifugation residence time. It has instead been shown that these increased levels arise from the changed state of the bacteria, which during digestion are viable but not measurable using standard plating techniques. Dewatering has the potential to reactivate or resuscitate the bacteria to make them measurable once again, as demonstrated using alternative methods (polymerase chain reaction) for bacterial analysis (Higgins et al., 2007(a)).
There is also some evidence (Qi et al., 2008) to suggest that regrowth in centrifuged biosolids is governed by the liquid to solid ratio (see also Sections 4−5).
3. Temperature effects
Reported E. coli concentrations in sludge at different temperatures for digestion and AD cake storage (Table 2) indicate a reduction in E. coli levels from AD, and regrowth during biosolids storage. Pathogen reduction from mesophilic anaerobic digestion (MAD) is predominantly due to substrate limitation (Rosenblum et al., 2014; Smith et al., 2005) rather than temperature, since E. coli growth rates are close to their optimal growth and survival temperature at the MAD operating temperatures (Lang and Smith, 2008).
Source | Waste Treatment | Temp | Effect on Pathogen Concentration |
---|---|---|---|
Le et al. (2002) | Municipal sludge secondary digestion | 28 °C | Pathogen deactivation, observed E. coli reduction of 1.7 Log g/DS |
Le et al. (2002) | Municipal sludge secondary digestion | 21 °C | Pathogen deactivation, observed E. coli reduction of 1.0 Log g/DS |
Iranpour et al. (2005) | Municipal sludge (thermophilic digestion) centrifuge outlet | 48.2 °C | Biosolids from dewatering centrifuge contained low number of faecal coliforms (1.9 Log g/DS) |
Iranpour et al. (2005) | Municipal sludge (thermophilic digestion) biosolids storage | 41 °C | Re-occurrence of faecal coliforms observed in silo storage (6.8 Log g/DS) |
Sprigings & Le (2011) | Municipal sludge biosolids storage (thermophilic digested) | 31 °C (at day 0) | |
28°C (at day 24) | E. coli concentration of 4.1 Log g/DS at day 0 of storage increasing to 5.9 Log g/DS at day 24 of storage | ||
Sprigings & Le (2011) | Municipal sludge biosolids storage (mesophilic digested) | 22.5 °C (at day 0) | |
20°C (at day 24) | E. coli concentration of 4 Log g/DS at day 0 of storage increasing to 5.1 Log g/DS at day 24 of storage |
The decreased temperatures following dewatering of the digested cake solids encourages regrowth of faecal coliforms (Iranpour et al., 2005). It has been suggested that a minimum temperature of 25 °C be maintained to prevent growth of faecal coliforms, based on evidence from operational field trials in digested and dewatered biosolids (Fane et al., 2020).
4. Moisture content effects
A decrease in the percent dry solids content (DS%) in dewatered biosolids may support prolonged cell survival, since there are more water-filled pores in the matrix which will increase nutrient availability to cells (Cools et al., 2001; Plachá et al., 2001; Semenov et al., 2007). This observation has been supported by a study of the dewatering of anaerobically digested biosolids by drying beds (Zaleski et al., 2005) which indicated a 1.5 order of magnitude increase in faecal coliform levels during a rainfall event which corresponded to a fall in the solids concentration to 20% dry solids (DS). This trend was also found for stored biosolids that were rewetted by rainfall (Rouch et al., 2011).
newsletterSignup is not templated
Against this, reported laboratory evidence (Lang and Smith, 2007) indicates that in dry, biosolids-amended soil, bacteria are protected within particles of sludge cake since drying restricts predatory activity. A marked increase in E. coli survival was observed in air-dried samples of sludge-amended soil. This observation has been supported by a study demonstrating the prolonged survival of a strain of E. coli in manure-amended soil even at moisture contents below 1% (Jiang et al., 2002).
The precise impact of moisture on pathogen survival in biosolids is thus conflicting. This perhaps reflects the heterogeneous nature of sludge itself, including nutrient and substrate availability and the indigenous organisms present, as well as the prevailing ambient conditions in large-scale studies.
5. Atmospheric effects
Mechanical dewatering causes substantial changes in the sludge cake environmental conditions with reference to oxygen availability.
It has been found (Qi et al., 2008; Erdal et al., 2003) that reactivation and regrowth is faster at increased cake dry solids concentrations. One explanation for this is the increased exposure to oxygen as centrifugal dewatering produces smaller flocs with an overall larger surface area (Chen et al., 2007).
Higher oxygen levels are detrimental to the survival of the obligate anaerobes from the anaerobic digestion process, creating a competitive advantage for the fast growing E. coli facultative anaerobes (Chen et al., 2007). The aerobic conditions may contribute to the higher level of indicator growth observed in the initial phase of storage, but this advantage is likely to recede as the storage conditions of biosolids return to an anaerobic state.
Controlling oxygen exposure during dewatering and subsequent storage may provide a means of suppressing E. coli post-digestion growth (Fig. 1), ensuring compliance targets are reliably met. A common practice for bacterial growth restriction used in agriculture is in silage production, which is the preservation of green foliage by acidification achieved through fermentation (Table 3). It may be possible for similar practices to be used to reduce indicator growth or enhance die-off rates in biosolids storage environments, where higher concentrations of pathogenic indicator bacteria during initial storage has been attributed to oxygen availability.
Microbial Control Mechanism | Source |
---|---|
Preservation method based on natural lactic acid fermentation of residual carbon under anaerobic conditions | Gollop et al., 2005; Herrmann et al., 2011 |
Compacting and sealing forage excludes air and enables physico-chemical and microbial change to occur in storage | Dunière et al., 2013 |
Growth and survival of pathogens depends on degree of anaerobiosis. Studies have shown that E. coli 0157:H7 does not survive a robust fermentation process | Avery et al., 2005; Bach et al., 2002; Byrne et al., 2002 |
Studies on the growth of E. coli 0157 in poorly fermented laboratory silage showed an increase from initial numbers of 103 E. coli 0157 CFU g-1 to numbers in excess of 106 CFU g-1 reflecting the ability of this organism to multiply rapidly in air-spoiled silage | Fenlon and Wilson 2000 |
Observations on the fate of E. coli during ensiling of wheat and corn showed that for wheat, samples inoculated with E. coli and sealed after a delay of 24 hours had less lactic acid and more acetic acid than other treatments. This may have been the cause of more prolonged activity in E. coli bacteria contained within these treatments as insufficient anaerobiosis can delay the lactic acid fermentation process, slowing pH decrease and increasing the survival of E. coli. High concentrations of E. coli were observed in decaying parts of silage, particularly in areas most susceptible to air penetration | Chen et al., 2005(b) |
The pH evolution plays an important role in silage preservation. Researchers observed E. coli strains surviving and growing only in silage showing a pH increase after samples were opened and had experienced 144 hours of aerobic exposure. Once exposed to oxygen, aerobic micro-organisms can develop and increase the pH levels in the remaining forage | Dunière et al., 2011 |
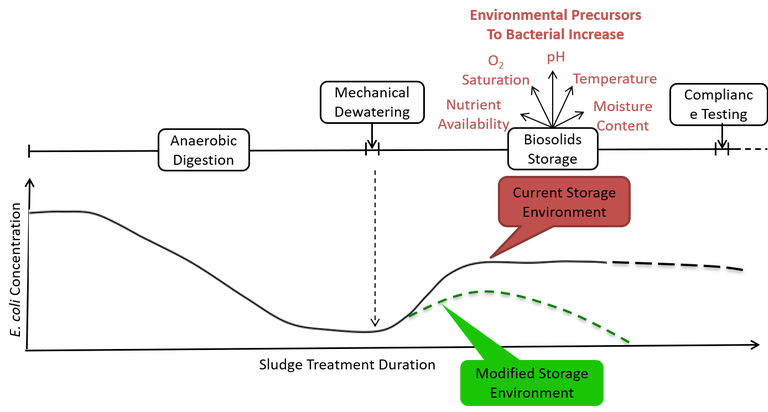
6. Summary
Research has identified high concentrations of indicator bacteria in anaerobically digested biosolids, following mechanical dewatering (Chen et al., 2011; Dentel et al., 2008; Higgins et al., 2006, 2007(b); Monteleone et al., 2004). A number of factors can be identified which influence E. coli growth and survival in the stored biosolids.
Firstly, it appears that mechanical dewatering processes may cause the release growth inducers, in the form of bioavailable organic matter, and transform the environmental conditions of the sludge matrix. Nutrient availability for cell survival and growth is a significant factor, along with the physical environmental conditions acting on cells held within stored biosolids.
The rigorous level of anaerobic digestion process control that precedes biosolids storage is likely to be largely negated by highly uncontrolled and poorly understood storage conditions in which external factors, including temperature and moisture content, may combine to preserve elevated indicator concentrations. The modified storage practice of ensiling may offer a viable method of inhibiting bacterial growth and survival, particularly when considered with external environmental parameter modifications.
As assurance of biosolids quality is increasingly sought by agriculturalists, retailers and the public, regulations and safety assurance schemes are becoming more stringent. At a time when water industry regulators worldwide are setting new market directions in waste trading, it is critical that the ambiguity concerning pathogen indicator concentrations is dispelled and sludge-to-land disposal routes are safeguarded.